Electrolysis is widely used in the manufacture of chemicals, facilitating the production of essential substances like chlorine, sodium hydroxide, and hydrogen through the controlled decomposition of ionic compounds in solution.
1) The chloralkali process
The chloralkali process is an electrolytic process for the manufacture of H2, Cl2 and NaOH.
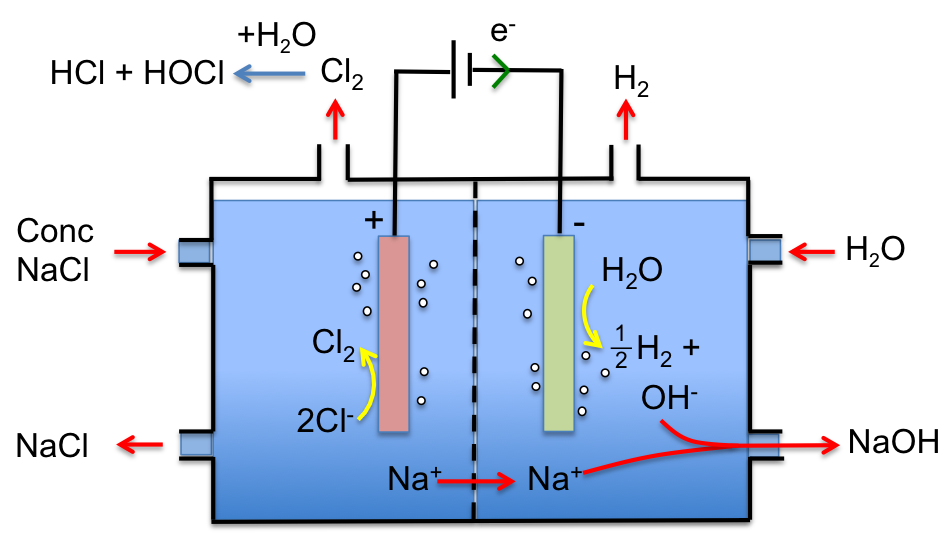
Concentrated NaCl (saturated brine) is pumped into the left compartment, and water is pumped into the right compartment. Chloride ions are oxidised at the inert titanium anode to form chlorine gas:
2Cl– (aq) → Cl2 (g) + 2e–
while water is reduced at the cathode to give hydrogen gas:
2H2O (l) + 2e– → H2 (g) + 2OH– (aq)
A cation exchange membrane, which allows sodium ions but not hydroxide and chloride ions to flow from the left compartment to the right compartment, is used. Hence, NaOH is only formed in the right compartment with the overall reaction being:
2NaCl (aq) + 2H2O (l) → Cl2 (g) + H2 (g) + 2NaOH (aq)
Chlorine gas, which is formed in the left compartment, is piped out, where it reacts with water to give hydrochloric and hypochlorous acids. Finally, NaCl can be replaced with KCl or CaCl2 for the manufacture of KOH or Ca(OH)2, respectively.

2) The Castner-Kellner process
Another way to manufacture H2, Cl2 and NaOH is via the Castner-Kellner process. The main difference between this process and the chloralkali process is the use of a liquid mercury cathode, which lowers the activation energy for the reduction of Na+ to Na, allowing the otherwise thermodynamically infeasible reaction to proceed.
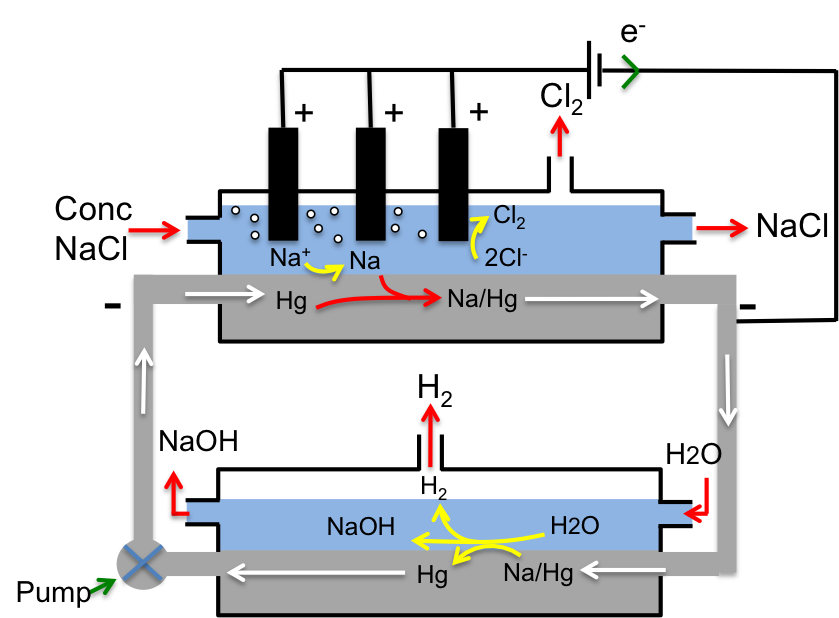
Upper compartment:
Concentrated NaCl is fed into the upper compartment where Cl– is oxidised at the inert titanium anode to liberate Cl2.
2Cl– (aq) → Cl2 (g) + 2e–
At the same time, Na+ is reduced at the Hg cathode to Na, which then dissolves in the cathode to form an amalgam, Na/Hg, which in turn circulates to the lower compartment.
Na+ (aq) + e– → Na (s)
Na (s) + Hg (l) → Na/Hg (l)
Lower compartment:
Water that is fed into the lower compartment reacts with the Na/Hg amalgam to give NaOH, H2 and Hg, which is pumped back up to the upper compartment for reuse. Note that Na is oxidised to Na+ while hydrogen in water is reduced to H2.
2Na/Hg (l) + 2H2O (l) → 2NaOH (aq) + H2 (g) + 2Hg (l)
The overall redox reaction is:
2NaCl (aq) + 2H2O (l) → 2NaOH (aq) + H2 (g) + 2Cl2 (g)